With new NIH support, Assistant Professor Michael Taylor explores the molecular signals that help build a protective layer between the brain and the rest of the body
By Jill Sakai
In the era of COVID-19, we’ve become accustomed to keeping our distance and using barriers to protect ourselves from potential threats in the environment. The vertebrate brain has long maintained its own version of this physical distancing with the blood-brain barrier, a tight layer of cells that lines blood vessels in the brain and restricts the free flow of molecules between the blood and the brain’s sensitive tissues.
This barrier plays a critical role in brain health, keeping out immune cells, toxins, pathogens, and other potential dangers. Unfortunately, the structure also prohibits the entry of many therapeutic agents into the brain, contributing to the difficulty in treating neurological disorders. But despite the blood-brain barrier’s importance, scientists don’t yet understand the molecular signals that help it form throughout the brain’s vasculature, or network of blood vessels — nor what leads it to break down in many diseases.
“The vasculature in the brain plays an important role in many diseases, including stroke, epilepsy, Alzheimer’s disease, and brain tumors,” says Michael Taylor, an assistant professor in the Drug Delivery Core of the Pharmaceutical Sciences Division in the University of Wisconsin–Madison School of Pharmacy.
“In many cases, the vessels lose the blood-brain barrier structure, which is important for normal brain function. In others, the blood-brain barrier actually makes it harder to get therapeutics where we need them,” he says. “So understanding these different signaling pathways and the crosstalk between them could have relevance to the treatment of many different neurological diseases.”
“If we understand what the signaling pathways are, then potentially we can repair a damaged blood-brain barrier in different disease states.”
—Michael Taylor
In April, Taylor was awarded a new five-year R01 grant from the National Institute of Neurological Diseases and Stroke to explore the interplay among the genetic factors that regulate the formation and function of the blood-brain barrier.
To learn more about how the brain’s vessels develop their barrier properties, he is comparing them with vessels found elsewhere in the body, which instead of a barrier have pores that allow the blood to exchange nutrients and waste products with muscles and organs.
“We know there are many different signal transduction pathways that are involved,” Taylor says. “The gist of our grant is to figure out the nuances of how these different pathways work together to make the vasculature in the brain different than the vasculature in the rest of the body.”
Following the molecular paths
Having the right organism is key to exploring the interplay between multiple complex signaling pathways. And here, Taylor says, zebrafish shine — literally. He has bred lines of these small striped fish to contain fluorescent tags that label individual proteins or specific populations of cells. With his powerful microscopy setup, his team can capture detailed images of the developing vasculature in an entire living zebrafish brain, even watching the process unfold in real time.
“Zebrafish provide a nice model system for doing this. The animals develop outside of the mother, so we can do time-lapse imaging and visualize all of this taking place in a live animal,” he says. What’s more, his team has bred fish to contain six or more added genes or markers so they can analyze multiple proteins and structures in the same animal.
These fish are helping the researchers tease apart the activity of different molecular signaling pathways. In a paper published in 2017, Taylor’s group showed that the blood vessels in the brain and the blood-brain barrier form simultaneously. But now they are learning that the two processes use different signaling mechanisms.
His lab group is focusing on two major biological pathways. One involves vascular endothelial growth factor, or VEGF, which guides blood vessel development in growing embryos as well as in cancerous tumors. The other is driven by the activity of two proteins called Wnt and beta-catenin, which play major roles in numerous important developmental processes, including early patterning of an animal’s basic body plan.
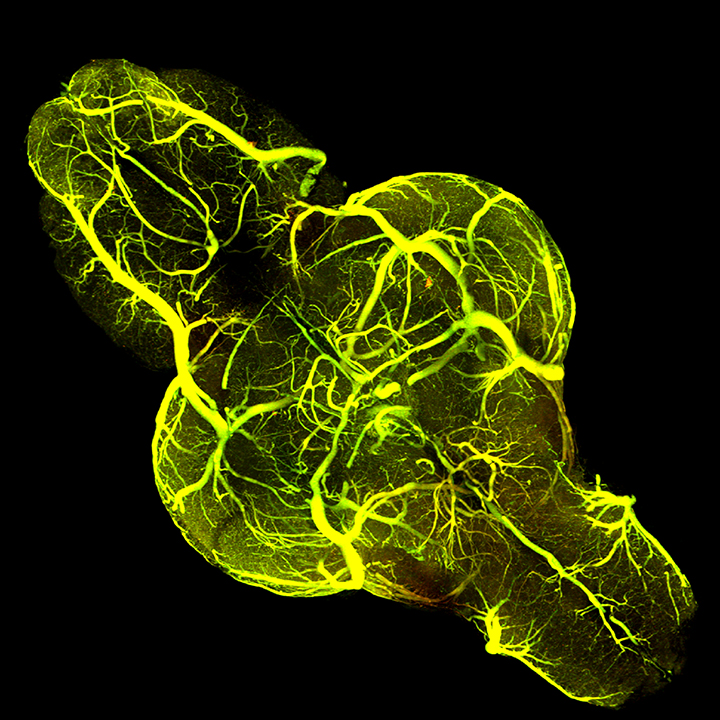
Taylor suspects that the two pathways functioning together are needed to allow blood vessels to grow in the brain and properly develop a blood-brain barrier. “Surprisingly, even though both of these pathways are very well studied, no one’s actually tried to link them together,” he says.
With their transgenic fish, Taylor’s team — including graduate students Annette Dean, Audrey Fetsko, and Dylan Sebo, and assistant scientist Kevin Lanham — can disrupt each pathway individually to see how it affects the growing blood vessels. For example, one strain of fish with a mutation in an essential Wnt receptor does not form either brain blood vessels or a blood-brain barrier, they have found. Another strain that has a mutation in a VEGF receptor also fails to form vessels in the brain but has other blood vessels that do acquire barrier properties.
“Because of that finding, we were able to say that both of these pathways are essential, but they’re not doing exactly the same thing,” Taylor says. Instead, he thinks that VEGF is primarily responsible for the vessel growth but that Wnt signaling plays dual roles: one in forming the blood-brain barrier, and one in regulating VEGF and, by extension, vessel growth in the brain.
Pathways to therapeutics
Other aspects of the research will look beyond the blood vessels to better understand how these same two pathways may interact with the broader brain environment.
Part of Wnt’s function in the endothelial cells lining the blood vessels has been known for 10 years, Taylor says, but the molecule is present in other cell types where its role is less clear but likely also important. One clue comes from a particular type of brain tumor called medulloblastoma, the most common brain cancer in children. Genetic mutations that mimic the activation of Wnt signaling in medulloblastoma cause defects in the blood-brain barrier within the tumor as it grows new vessels.
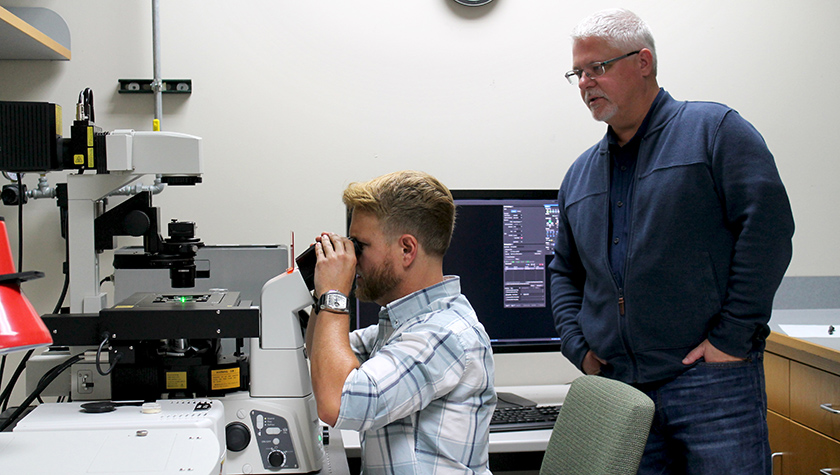
With their zebrafish model system, Taylor’s group can alter Wnt signaling in different cell types during development. So far, they’ve found that Wnt activation in a type of neural progenitor cell called radial glia — but, surprisingly, not in neurons themselves — can completely block vessel formation in the brain. With the new funding, they will work to identify key molecules that help drive these distinct effects.
Understanding the full range of potential effects is important from a therapeutic perspective. Wnt signaling is an appealing possible drug target because its role in blood vessel growth appears to be very specific to the brain, he says. “It may have the intended effect on endothelial cells but could also have very profound effects on other cell types in the brain,” he says. “We’re trying to figure out the consequences of activating or inhibiting this pathway in the brain in general, not just in endothelial cells.”
In other diseases, such as neurodegenerative disorders, the vessels lose their barrier structure and become leaky. In some ways, they start to resemble the vessels elsewhere in the body with their pores for exchanging nutrients. A third aim of Taylor’s grant is to look at what regulates whether a vessel will develop those pores. So far, he says, very little is known about this process, but VEGF seems to be involved.
“If we understand what the signaling pathways are, then potentially we can repair a damaged blood-brain barrier in different disease states,” Taylor says. “Currently, there is absolutely no treatment strategy for that kind of problem.”